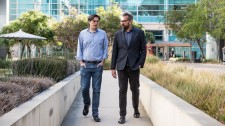
A new study uses large-scale genetic approach to uncover human genes controlling HIV infection.
SAN FRANCISCO, February 20, 2020 (Newswire.com) - Viruses are parasites. The only way they can grow is by hijacking their hosts. When they infect a human host, viruses use human proteins to multiply and modify the human cells to sustain the infection. At the same time, the human host activates defense mechanisms to fight the infection.
Most current drugs against viral infections target the virus itself. But scientists are interested in developing therapies that aim for host proteins instead, or the genes that produce them, in part because such therapies are believed less likely to elicit drug resistance. A detailed understanding of virus-host interactions is crucial to the success of this strategy.
A team of Gladstone Institutes scientists, led by Senior Investigator Nevan Krogan, PhD, has been cataloging host proteins that physically bind to virus proteins. These physical interactions identify human proteins that the virus can use to infect cells and propagate. However, they don’t reveal how host proteins work together to facilitate infection.
To address this gap, Krogan and staff scientist David Gordon, PhD, with colleagues at UC San Francisco (UCSF), University College Dublin, and the Mount Sinai School of Medicine, have developed a new way to understand how host cells control HIV infection in human cells.
Their approach entails disrupting host genes rather than proteins. It is based on the idea, pioneered by Krogan, that you obtain richer information about the functions of genes—and the proteins they encode—when you disable the genes in pairs, instead of one by one. In a paper published in Molecular Cell, the team describes a map of the genes controlling HIV infection in human cells, which they built by assessing more than 63,000 combinations of human genes associated with HIV infection.
“HIV is a major public health concern, with an estimated 36.7 million people living with chronic infection and over 20.9 million people receiving continuous treatment,” said Krogan, who is also a professor of cellular and molecular pharmacology at UCSF and the director of the UCSF Quantitative Biosciences Institute. “Studying the impact of gene disruptions in pairs, rather than one by one, yields important information on how genes work together to mediate virus infection, highlighting processes we can target with drugs to inhibit infection.”
The map, which the team refers to as a viral epistasis map (vE-MAP), is an essential advancement for HIV research in several other ways. For one thing, it uncovers a previously unsuspected set of genes required for the growth of the virus in human cells. For another, the vE-MAP can be used to analyze how different HIV mutants affect host cells or to test drugs that disrupt HIV-host interactions.
Strength in Numbers
The vE-MAP is an adaptation of the E-MAP, which Krogan and his colleagues pioneered and refined over the past 15 years to identify genes that control how cells grow. At the core of this approach is their ability to disrupt a large number of genes, test them in pairs, and analyze the results via sophisticated computational methods.
“The principle behind E-MAPs is that when you disrupt two genes at once and examine the impact on a cell, you sometimes see effects that are significantly larger or smaller than you would have predicted from the effect of disrupting either gene alone,” said Krogan.
These unexpected effects suggest that the functions of the two genes are related. Moreover, by carrying out these pairwise disruptions across hundreds of genes, scientists can find groups of genes with similar patterns of interactions, a sign that they are likely to take part in the same molecular process.
“And so, instead of finding important genes one at a time, you can all at once identify multiple, distinct networks of genes affecting the process you are studying,” said Gordon.
The E-MAP approach has mostly been used to study cell growth. Gordon, in collaboration with a student from University College Dublin, Ariane Watson, had to modify it to study virus infection. The most tricky part was to implement a sophisticated data acquisition and scoring system, which allowed them to measure HIV infection accurately across hundreds of thousands of samples, and compare the effect of pairwise and single-gene disruptions.
It would be an overwhelming effort to test all combinations of the over 20,000 protein-coding genes in the human genome. Instead, the scientists focused on genes already suspected to influence HIV biology. In particular, they used the genes encoding a large number of human proteins that Krogan's lab had previously found to bind to HIV proteins. In all, they included over 350 genes in their analysis and tested over 63,000 pairwise disruptions.
New Players at the HIV-Host Interface
Although HIV is one of the best-studied human viruses and is now well-controlled by antiretroviral therapy, there is no cure for HIV/AIDS. Moreover, antiretroviral therapy is costly, which can make it impractical in resource-poor countries. The search for new means of halting or eradicating the virus is therefore still a priority.
Among the genes that stood out in the vE-MAP were several members of the CNOT family, whose role in HIV biology had never before been established. The authors demonstrated that the CNOT complex promotes HIV infection by suppressing innate immunity in CD4+ T cells, the type of immune cells that HIV preferentially targets in humans. Innate immunity is a defense mechanism by which host cells can fight infection.
“The impact of CNOT on innate immunity is a key, yet previously unrecognized, host pathway critical to HIV infection. It will serve as a potential novel therapeutic target in future studies,” said Krogan.
For instance, scientists can now study if targeting the CNOT complex with drugs could be a way to help HIV patients fight the infection more effectively.
Furthermore, the vE-MAP uncovered genes that had little impact when disrupted individually but had great effect when tested together.
“These genes would be overlooked in classic, single-gene disruption experiments,” said Gordon. “They confirm the potential of the vE-MAP to uncover new mechanisms by which HIV interacts with human cells.”
Combining drugs that target two of these genes at the same time might thus be a promising therapeutic strategy, especially for a virus such as HIV/AIDS, which has evolved multiple ways of tapping its hosts’ resources.
The vE-MAP was also able to pick up genes that specifically interact with a known HIV mutant. This observation bodes well for the ability of the vE-MAP to identify distinct host factors affecting the various forms of HIV, or the virus mutants that arise in response to currently available drugs.
Additional testing with a drug known to interfere with HIV-associated human proteins gives the authors confidence that their vE-MAP approach could in the future be used to screen for novel anti-HIV drugs and to understand their mode of action.
“This vE-MAP provides an unprecedented view of how HIV hijacks and rewires the cellular machinery in human cells during infection,” said Krogan. “It will generate many new ideas and avenues to identify and test novel therapies.”
And the benefits may not be limited to HIV research.
“Our work is proof-of-principle that the vE-MAP approach is a powerful way to map out the interface between HIV and human cells, and to uncover new therapeutic avenues,” said Gordon. “We now look forward to testing it on other pathogens.”
About the Research Project
Other authors include: Erica Stevenson, Danielle L. Swaney, and Alexander Marson from Gladstone Institutes; Assen Roguev, Gwendolyn M. Jang, Joshua Kane, Jiewei Xu, Jeffrey Z. Guo, Kathy Franks-Skiba, Michael Shales, David C. Crosby, and Alan D. Frankel from UC San Francisco; Ariane Watson and Gerard Cagney from University College Dublin, Ireland; and Simin Zheng and Ivan Marazzi from Icahn School of Medicine at Mount Sinai, New York.
This work was supported by an NIH Postdoctoral Fellowship (5F32GM108303-02), the HARC Center (P50 AI150476), the Host Pathogen Map Initiative (U19 AI135990), the FluOMICS consortia (U19 AI135972), and a Science Foundation Ireland Principal Investigator Award (SFI 10/1N.1/B3.19). Additional funding came from the European Union’s Horizon 2020 (grant agreement 666010), NIH grants P30 AI027763 and S10 RR028962, and the James B. Pendleton Charitable Trust.
Media Contact: Megan McDevitt
Megan.mcdevitt@Gladstone.ucsf.edu
415.734.2019
Source: Gladstone Institutes
Share: